Dynamic regulation of transcriptional states by chromatin and transcription factors
The interaction of regulatory proteins with the complex nucleoprotein structures that are found in mammalian cells involves chromatin reorganization at multiple levels. Mechanisms that support these transitions are complex on many timescales, which range from milliseconds to minutes or hours. In this Review, we discuss emerging concepts regarding the function of regulatory elements in living cells. We also explore the involvement of these dynamic and stochastic processes in the evolution of fluctuating transcriptional activity states that are now commonly reported in eukaryotic systems.
This is a preview of subscription content, access via your institution
Access options
Subscribe to this journal
Receive 12 print issues and online access
206,07 € per year
only 17,17 € per issue
Buy this article
- Purchase on SpringerLink
- Instant access to full article PDF
Prices may be subject to local taxes which are calculated during checkout
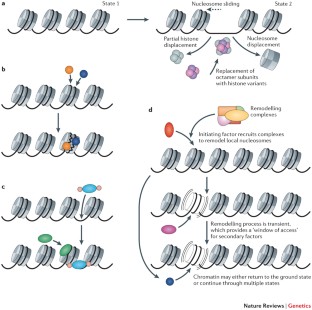
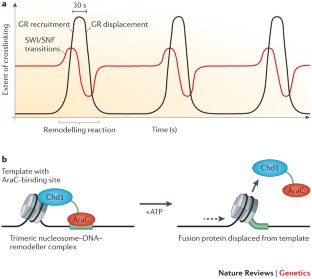
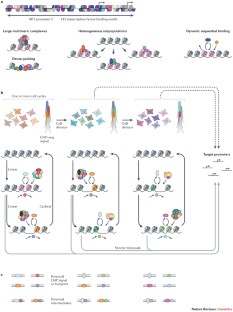
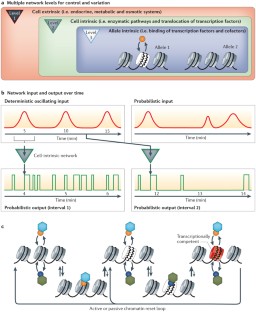
Similar content being viewed by others
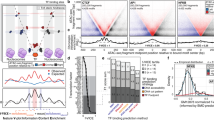
Chromatin information content landscapes inform transcription factor and DNA interactions
Article Open access 26 February 2021
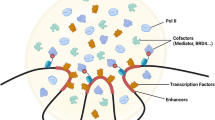
Emerging insights into transcriptional condensates
Article Open access 25 April 2024
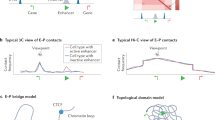
The spatial organization of transcriptional control
Article 14 September 2022
References
- Johnson, D. G. & Dent, S. Y. Chromatin: receiver and quarterback for cellular signals. Cell152, 685–689 (2013). CASPubMedPubMed CentralGoogle Scholar
- Hardison, R. C. & Taylor, J. Genomic approaches towards finding cis-regulatory modules in animals. Nature Rev. Genet.13, 469–483 (2012). CASPubMedGoogle Scholar
- Baylin, S. B. & Jones, P. A. A decade of exploring the cancer epigenome — biological and translational implications. Nature Rev. Cancer11, 726–734 (2011). CASGoogle Scholar
- Spitz, F. & Furlong, E. E. Transcription factors: from enhancer binding to developmental control. Nature Rev. Genet.13, 613–626 (2012). CASPubMedGoogle Scholar
- Wu, W. et al. Dynamics of the epigenetic landscape during erythroid differentiation after GATA1 restoration. Genome Res.21, 1659–1671 (2011). CASPubMedPubMed CentralGoogle Scholar
- Reik, W. Stability and flexibility of epigenetic gene regulation in mammalian development. Nature447, 425–432 (2007). CASPubMedGoogle Scholar
- Spivakov, M. & Fisher, A. G. Epigenetic signatures of stem-cell identity. Nature Rev. Genet.8, 263–271 (2007). CASPubMedGoogle Scholar
- Rivera, C. M. & Ren, B. Mapping human epigenomes. Cell155, 39–55 (2013). CASPubMedGoogle Scholar
- Thurman, R. E. et al. The accessible chromatin landscape of the human genome. Nature489, 75–82 (2012). CASPubMedPubMed CentralGoogle Scholar
- Degner, J. F. et al. DNase I sensitivity QTLs are a major determinant of human expression variation. Nature482, 390–394 (2012). CASPubMedPubMed CentralGoogle Scholar
- Sanyal, A., Lajoie, B. R., Jain, G. & Dekker, J. The long-range interaction landscape of gene promoters. Nature489, 109–113 (2012). CASPubMedPubMed CentralGoogle Scholar
- Larson, D. R. What do expression dynamics tell us about the mechanism of transcription? Curr. Opin. Genet. Dev.21, 591–599 (2011). CASPubMedPubMed CentralGoogle Scholar
- Mellor, J. Dynamic nucleosomes and gene transcription. Trends Genet.22, 320–329 (2006). CASPubMedGoogle Scholar
- McNally, J. G., Mueller, W. G., Walker, D., Wolford, R. G. & Hager, G. L. The glucocorticoid receptor: rapid exchange with regulatory sites in living cells. Science287, 1262–1265 (2000). This paper reports the first observation of site-specific factor binding to a regulatory element in living cells, which reveals rapid exchange dynamics.CASPubMedGoogle Scholar
- Kaern, M., Elston, T. C., Blake, W. J. & Collins, J. J. Stochasticity in gene expression: from theories to phenotypes. Nature Rev. Genet.6, 451–464 (2005). CASPubMedGoogle Scholar
- Coulon, A., Chow, C. C., Singer, R. H. & Larson, D. R. Eukaryotic transcriptional dynamics: from single molecules to cell populations. Nature Rev. Genet.14, 572–584 (2013). CASPubMedGoogle Scholar
- Boyle, A. P. et al. High-resolution mapping and characterization of open chromatin across the genome. Cell132, 311–322 (2008). CASPubMedPubMed CentralGoogle Scholar
- John, S. et al. Chromatin accessibility pre-determines glucocorticoid receptor binding patterns. Nature Genet.43, 264–268 (2011). CASPubMedGoogle Scholar
- Hoogenkamp, M. et al. Early chromatin unfolding by RUNX1: a molecular explanation for differential requirements during specification versus maintenance of the hematopoietic gene expression program. Blood114, 299–309 (2009). CASPubMedPubMed CentralGoogle Scholar
- Siersbaek, R. et al. Extensive chromatin remodelling and establishment of transcription factor 'hotspots' during early adipogenesis. EMBO J.30, 1459–1472 (2011). CASPubMedPubMed CentralGoogle Scholar
- Stamatoyannopoulos, J. A. et al. An encyclopedia of mouse DNA elements (mouse ENCODE). Genome Biol.13, 418 (2012). PubMedPubMed CentralGoogle Scholar
- Biddie, S. C. et al. Transcription factor AP1 potentiates chromatin accessibility and glucocorticoid receptor binding. Mol. Cell43, 145–155 (2011). CASPubMedPubMed CentralGoogle Scholar
- Heintzman, N. D. & Ren, B. Finding distal regulatory elements in the human genome. Curr. Opin. Genet. Dev.19, 541–549 (2009). CASPubMedPubMed CentralGoogle Scholar
- Bosisio, D. et al. A hyper-dynamic equilibrium between promoter-bound and nucleoplasmic dimers controls NF-κB-dependent gene activity. EMBO J.25, 798–810 (2006). CASPubMedPubMed CentralGoogle Scholar
- Sharp, Z. D. et al. Estrogen-receptor-α exchange and chromatin dynamics are ligand-and domain-dependent. J. Cell Sci.119, 4101–4116 (2006). CASPubMedGoogle Scholar
- Yao, J., Munson, K. M., Webb, W. W. & Lis, J. T. Dynamics of heat shock factor association with native gene loci in living cells. Nature442, 1050–1053 (2006). CASPubMedGoogle Scholar
- Lickwar, C. R., Mueller, F., Hanlon, S. E., McNally, J. G. & Lieb, J. D. Genome-wide protein–DNA binding dynamics suggest a molecular clutch for transcription factor function. Nature484, 251–255 (2012). CASPubMedPubMed CentralGoogle Scholar
- Kuipers, M. A. et al. Highly stable loading of Mcm proteins onto chromatin in living cells requires replication to unload. J. Cell Biol.192, 29–41 (2011). CASPubMedPubMed CentralGoogle Scholar
- Stasevich, T. J. & McNally, J. G. Assembly of the transcription machinery: ordered and stable, random and dynamic, or both? Chromosoma120, 533–545 (2011). CASPubMedPubMed CentralGoogle Scholar
- Mueller, F., Mazza, D., Stasevich, T. J. & McNally, J. G. FRAP and kinetic modeling in the analysis of nuclear protein dynamics: what do we really know? Curr. Opin. Cell Biol.22, 403–411 (2010). CASPubMedPubMed CentralGoogle Scholar
- Neph, S. et al. An expansive human regulatory lexicon encoded in transcription factor footprints. Nature489, 83–90 (2012). This study carries out an exhaustive characterization of DNaseI hypersensitive regions in human cell lines, which reveals the presence of many transcription factors by their footprints within the accessible region.CASPubMedPubMed CentralGoogle Scholar
- Deng, W. et al. Controlling long-range genomic interactions at a native locus by targeted tethering of a looping factor. Cell149, 1233–1244 (2012). This study finds that LIM domain-binding protein 1(LDB1)effects GATA1-mediated chromatin loop formation by long-range protein–protein interactions.CASPubMedPubMed CentralGoogle Scholar
- Stender, J. D. et al. Genome-wide analysis of estrogen receptor α DNA binding and tethering mechanisms identifies Runx1 as a novel tethering factor in receptor-mediated transcriptional activation. Mol. Cell. Biol.30, 3943–3955 (2010). CASPubMedPubMed CentralGoogle Scholar
- Erdel, F., Krug, J., Langst, G. & Rippe, K. Targeting chromatin remodelers: signals and search mechanisms. Biochim. Biophys. Acta1809, 497–508 (2011). CASPubMedGoogle Scholar
- Cairns, B. R. Chromatin remodeling: insights and intrigue from single-molecule studies. Nature Struct. Mol. Biol.14, 989–996 (2007). CASGoogle Scholar
- Clapier, C. R. & Cairns, B. R. The biology of chromatin remodeling complexes. Annu. Rev. Biochem.78, 273–304 (2009). CASPubMedGoogle Scholar
- Glatt, S., Alfieri, C. & Muller, C. W. Recognizing and remodeling the nucleosome. Curr. Opin. Struct. Biol.21, 335–341 (2011). CASPubMedGoogle Scholar
- Hargreaves, D. C. & Crabtree, G. R. ATP-dependent chromatin remodeling: genetics, genomics and mechanisms. Cell Res.21, 396–420 (2011). CASPubMedPubMed CentralGoogle Scholar
- Korber, P. & Becker, P. B. Nucleosome dynamics and epigenetic stability. Essays Biochem.48, 63–74 (2010). CASPubMedGoogle Scholar
- Miller, J. A. & Widom, J. Collaborative competition mechanism for gene activation in vivo. Mol. Cell. Biol.23, 1623–1632 (2003). CASPubMedPubMed CentralGoogle Scholar
- Mirny, L. A. Nucleosome-mediated cooperativity between transcription factors. Proc. Natl Acad. Sci. USA107, 22534–22539 (2010). CASPubMedPubMed CentralGoogle Scholar
- Teif, V. B., Ettig, R. & Rippe, K. A lattice model for transcription factor access to nucleosomal DNA. Biophys. J.99, 2597–2607 (2010). CASPubMedPubMed CentralGoogle Scholar
- Zaret, K. S. & Carroll, J. S. Pioneer transcription factors: establishing competence for gene expression. Genes Dev.25, 2227–2241 (2011). CASPubMedPubMed CentralGoogle Scholar
- Hatta, M. & Cirillo, L. A. Chromatin opening and stable perturbation of core histone: DNA contacts by FoxO1. J. Biol. Chem.282, 35583–35593 (2007). CASPubMedGoogle Scholar
- Magnani, L., Eeckhoute, J. & Lupien, M. Pioneer factors: directing transcriptional regulators within the chromatin environment. Trends Genet.27, 465–474 (2011). CASPubMedGoogle Scholar
- Lupien, M. et al. FoxA1 translates epigenetic signatures into enhancer-driven lineage-specific transcription. Cell132, 958–970 (2008). CASPubMedPubMed CentralGoogle Scholar
- Jozwik, K. M. & Carroll, J. S. Pioneer factors in hormone-dependent cancers. Nature Rev. Cancer12, 381–385 (2012). CASGoogle Scholar
- Cirillo, L. A. & Zaret, K. S. Specific interactions of the wing domains of FOXA1 transcription factor with DNA. J. Mol. Biol.366, 720–724 (2007). In this study, FOXA1 is characterized as a pioneer protein through site-specific interactions with DNA.CASPubMedGoogle Scholar
- Boeger, H., Griesenbeck, J., Strattan, J. S. & Kornberg, R. D. Removal of promoter nucleosomes by disassembly rather than sliding in vivo. Mol. Cell14, 667–673 (2004). CASPubMedGoogle Scholar
- Boeger, H., Griesenbeck, J. & Kornberg, R. D. Nucleosome retention and the stochastic nature of promoter chromatin remodeling for transcription. Cell133, 716–726 (2008). This paper presents nucleosome occupancy at the yeastPHO5locus as a dynamic equilibrium between multiple states.CASPubMedPubMed CentralGoogle Scholar
- Nagaich, A. K., Walker, D. A., Wolford, R. G. & Hager, G. L. Rapid periodic binding and displacement of the glucocorticoid receptor during chromatin remodeling. Mol. Cell14, 163–174 (2004). This study uses UV laser crosslinking to characterize dynamics of factor binding during chromatin remodelling.CASPubMedGoogle Scholar
- McKnight, J. N., Jenkins, K. R., Nodelman, I. M., Escobar, T. & Bowman, G. D. Extranucleosomal DNA binding directs nucleosome sliding by chd1. Mol. Cell. Biol.31, 4746–4759 (2011). This paper shows that nucleosome invasion of a factor-specific binding site, which is catalysed by a remodelling protein, displaces the binding complex from the site.CASPubMedPubMed CentralGoogle Scholar
- Kassabov, S. R., Henry, N. M., Zofall, M., Tsukiyama, T. & Bartholomew, B. High-resolution mapping of changes in histone–DNA contacts of nucleosomes remodeled by ISW2. Mol. Cell. Biol.22, 7524–7534 (2002). CASPubMedPubMed CentralGoogle Scholar
- Voss, T. C. et al. Dynamic exchange at regulatory elements during chromatin remodeling underlies assisted loading mechanism. Cell146, 544–554 (2011). This study shows that two factors that bind to the same DNA recognition element fail to manifest competition in living cells, which leads to the hypothesis of dynamic assisted loading.CASPubMedPubMed CentralGoogle Scholar
- MacArthur, S. et al. Developmental roles of 21 Drosophila transcription factors are determined by quantitative differences in binding to an overlapping set of thousands of genomic regions. Genome Biol.10, R80 (2009). PubMedPubMed CentralGoogle Scholar
- Moorman, C. et al. Hotspots of transcription factor colocalization in the genome of Drosophila melanogaster. Proc. Natl Acad. Sci. USA103, 12027–12032 (2006). This paper shows that theDrosophila melanogastergenome contains many hot spots that are targeted by multiple transcription factors.CASPubMedPubMed CentralGoogle Scholar
- Zinzen, R. P., Girardot, C., Gagneur, J., Braun, M. & Furlong, E. E. Combinatorial binding predicts spatio-temporal cis-regulatory activity. Nature462, 65–70 (2009). CASPubMedGoogle Scholar
- Farnham, P. J. Insights from genomic profiling of transcription factors. Nature Rev. Genet.10, 605–616 (2009). CASPubMedGoogle Scholar
- modENCODE Consortium et al. Identification of functional elements and regulatory circuits by Drosophila modENCODE. Science330, 1787–1797 (2010).
- Grandori, C., Cowley, S. M., James, L. P. & Eisenman, R. N. The Myc/Max/Mad network and the transcriptional control of cell behavior. Annu. Rev. Cell Dev. Biol.16, 653–699 (2000). CASPubMedGoogle Scholar
- Rigaud, G., Roux, J., Pictet, R. & Grange, T. In vivo footprinting of rat TAT gene: dynamic interplay between the glucocorticoid receptor and a liver-specific factor. Cell67, 977–986 (1991). This paper shows that the glucocorticoid receptor induces binding of a liver transcription factor upstream of the tyrosine aminotransferase gene (Tat), even though the receptor and the liver factor compete for binding as pure proteins on naked DNA.CASPubMedGoogle Scholar
- Morris, S. A. et al. Overlapping chromatin-remodeling systems collaborate genome wide at dynamic chromatin transitions. Nature Struct. Mol. Biol.http://dx.doi.org/10.1038/nsmb.2718 (2013).
- Hilfinger, A. & Paulsson, J. Separating intrinsic from extrinsic fluctuations in dynamic biological systems. Proc. Natl Acad. Sci. USA108, 12167–12172 (2011). CASPubMedPubMed CentralGoogle Scholar
- Swain, P. S., Elowitz, M. B. & Siggia, E. D. Intrinsic and extrinsic contributions to stochasticity in gene expression. Proc. Natl Acad. Sci. USA99, 12795–12800 (2002). CASPubMedPubMed CentralGoogle Scholar
- Harper, C. V. et al. Dynamic analysis of stochastic transcription cycles. PLoS. Biol.9, e1000607 (2011). CASPubMedPubMed CentralGoogle Scholar
- John, S. et al. Kinetic complexity of the global response to glucocorticoid receptor action. Endocrinology150, 1766–1774 (2009). CASPubMedPubMed CentralGoogle Scholar
- Voss, T. C., John, S. & Hager, G. L. Single cell analysis of glucocorticoid receptor action reveals that stochastic post-chromatin association mechanisms regulate ligand-specific transcription. Mol. Endocrinol.20, 2641–2655 (2006). CASPubMedGoogle Scholar
- Elowitz, M. B., Levine, A. J., Siggia, E. D. & Swain, P. S. Stochastic gene expression in a single cell. Science297, 1183–1186 (2002). CASPubMedGoogle Scholar
- Raser, J. M. & O'Shea, E. K. Control of stochasticity in eukaryotic gene expression. Science304, 1811–1814 (2004). CASPubMedPubMed CentralGoogle Scholar
- Alon, U. Network motifs: theory and experimental approaches. Nature Rev. Genet.8, 450–461 (2007). CASPubMedGoogle Scholar
- Yosef, N. & Regev, A. Impulse control: temporal dynamics in gene transcription. Cell144, 886–896 (2011). CASPubMedPubMed CentralGoogle Scholar
- Veldhuis, J. D., Keenan, D. M. & Pincus, S. M. Motivations and methods for analyzing pulsatile hormone secretion. Endocr. Rev.29, 823–864 (2008). CASPubMedPubMed CentralGoogle Scholar
- Stavreva, D. A. et al. Ultradian hormone stimulation induces glucocorticoid receptor-mediated pulses of gene transcription. Nature Cell Biol.11, 1093–1102 (2009). CASPubMedGoogle Scholar
- Stavreva, D. A., Varticovski, L. & Hager, G. L. Complex dynamics of transcription regulation. Biochim. Biophys. Acta1819, 657–666 (2012). CASPubMedPubMed CentralGoogle Scholar
- Hughes, M. E. et al. Harmonics of circadian gene transcription in mammals. PLoS. Genet.5, e1000442 (2009). PubMedPubMed CentralGoogle Scholar
- Vollmers, C. et al. Time of feeding and the intrinsic circadian clock drive rhythms in hepatic gene expression. Proc. Natl Acad. Sci. USA106, 21453–21458 (2009). CASPubMedPubMed CentralGoogle Scholar
- Ou, Q., Magico, A. & King-Jones, K. Nuclear receptor DHR4 controls the timing of steroid hormone pulses during Drosophila development. PLoS. Biol.9, e1001160 (2011). CASPubMedPubMed CentralGoogle Scholar
- Uhlendorf, J. et al. Long-term model predictive control of gene expression at the population and single-cell levels. Proc. Natl Acad. Sci. USA109, 14271–14276 (2012). CASPubMedPubMed CentralGoogle Scholar
- Hoffmann, A. & Baltimore, D. Circuitry of nuclear factor κB signaling. Immunol. Rev.210, 171–186 (2006). PubMedGoogle Scholar
- Sung, M. H. et al. Sustained oscillations of NF-κB produce distinct genome scanning and gene expression profiles. PLoS ONE4, e7163 (2009). PubMedPubMed CentralGoogle Scholar
- Nelson, D. E. et al. Oscillations in NF-κB signaling control the dynamics of gene expression. Science306, 704–708 (2004). CASPubMedGoogle Scholar
- Lahav, G. et al. Dynamics of the p53–Mdm2 feedback loop in individual cells. Nature Genet.36, 147–150 (2004). This study shows that p53 is expressed in discrete pulses after DNA damage and that the number, but not the size, of pulses increases with the extent of DNA damage.CASPubMedGoogle Scholar
- Ashall, L. et al. Pulsatile stimulation determines timing and specificity of NF-κB-dependent transcription. Science324, 242–246 (2009). CASPubMedPubMed CentralGoogle Scholar
- Purvis, J. E. et al. p53 dynamics control cell fate. Science336, 1440–1444 (2012). CASPubMedPubMed CentralGoogle Scholar
- Bratsun, D., Volfson, D., Tsimring, L. S. & Hasty, J. Delay-induced stochastic oscillations in gene regulation. Proc. Natl Acad. Sci. USA102, 14593–14598 (2005). CASPubMedPubMed CentralGoogle Scholar
- Cai, L., Dalal, C. K. & Elowitz, M. B. Frequency-modulated nuclear localization bursts coordinate gene regulation. Nature455, 485–490 (2008). CASPubMedPubMed CentralGoogle Scholar
- Hao, N. & O'Shea, E. K. Signal-dependent dynamics of transcription factor translocation controls gene expression. Nature Struct. Mol. Biol.19, 31–39 (2012). CASGoogle Scholar
- Blake, W. J., Kaern, M., Cantor, C. R. & Collins, J. J. Noise in eukaryotic gene expression. Nature422, 633–637 (2003). CASPubMedGoogle Scholar
- Janicki, S. M. et al. From silencing to gene expression: real-time analysis in single cells. Cell116, 683–698 (2004). CASPubMedPubMed CentralGoogle Scholar
- Golding, I., Paulsson, J., Zawilski, S. M. & Cox, E. C. Real-time kinetics of gene activity in individual bacteria. Cell123, 1025–1036 (2005). CASPubMedGoogle Scholar
- Chubb, J. R., Trcek, T., Shenoy, S. M. & Singer, R. H. Transcriptional pulsing of a developmental gene. Curr. Biol.16, 1018–1025 (2006). CASPubMedPubMed CentralGoogle Scholar
- Raj, A., Peskin, C. S., Tranchina, D., Vargas, D. Y. & Tyagi, S. Stochastic mRNA synthesis in mammalian cells. PLoS. Biol.4, e309 (2006). PubMedPubMed CentralGoogle Scholar
- Darzacq, X. et al. In vivo dynamics of RNA polymerase II transcription. Nature Struct. Mol. Biol.14, 796–806 (2007). CASGoogle Scholar
- Suter, D. M., Molina, N., Naef, F. & Schibler, U. Origins and consequences of transcriptional discontinuity. Curr. Opin. Cell Biol.23, 657–662 (2011). CASPubMedGoogle Scholar
- Wilkinson, D. J. Stochastic modelling for quantitative description of heterogeneous biological systems. Nature Rev. Genet.10, 122–133 (2009). CASPubMedGoogle Scholar
- Kim, H. D., Shay, T., O'Shea, E. K. & Regev, A. Transcriptional regulatory circuits: predicting numbers from alphabets. Science325, 429–432 (2009). CASPubMedPubMed CentralGoogle Scholar
- Ko, M. S., Nakauchi, H. & Takahashi, N. The dose dependence of glucocorticoid-inducible gene expression results from changes in the number of transcriptionally active templates. EMBO J.9, 2835–2842 (1990). This paper shows that induction of gene expression by the glucocorticoid receptor results from increases in the frequency of active templates but not from the extent of transcription from each template.CASPubMedPubMed CentralGoogle Scholar
- Archer, T. K. et al. Differential steroid hormone induction of transcription from the mouse mammary tumor virus promoter. Mol. Endocrinol.8, 568–576 (1994). CASPubMedGoogle Scholar
- Becker, M. et al. Dynamic behavior of transcription factors on a natural promoter in living cells. EMBO Rep.3, 1188–1194 (2002). CASPubMedPubMed CentralGoogle Scholar
- Shang, Y., Hu, X., DiRenzo, J., Lazar, M. A. & Brown, M. Cofactor dynamics and sufficiency in estrogen receptor-regulated transcription. Cell103, 843–852 (2000). CASPubMedGoogle Scholar
- Metivier, R. et al. Estrogen receptor-α directs ordered, cyclical, and combinatorial recruitment of cofactors on a natural target promoter. Cell115, 751–763 (2003). CASPubMedGoogle Scholar
- Hah, N. et al. A rapid, extensive, and transient transcriptional response to estrogen signaling in breast cancer cells. Cell145, 622–634 (2011). CASPubMedPubMed CentralGoogle Scholar
- Karpova, T. S. et al. Concurrent fast and slow cycling of a transcriptional activator at an endogenous promoter. Science319, 466–469 (2008). CASPubMedGoogle Scholar
- Magklara, A. et al. An epigenetic signature for monoallelic olfactory receptor expression. Cell145, 555–570 (2011). CASPubMedPubMed CentralGoogle Scholar
- Suter, D. M. et al. Mammalian genes are transcribed with widely different bursting kinetics. Science332, 472–474 (2011). This study expresses a luciferase protein that is detected by bioluminescence in single cells, which reveals bursting kinetics that is characterized by refractory and active periods.CASPubMedGoogle Scholar
- Voss, T. C. et al. Combinatorial probabilistic chromatin interactions produce transcriptional heterogeneity. J. Cell. Sci.122, 345–356 (2009). CASPubMedPubMed CentralGoogle Scholar
- McFerran, D. W. et al. Persistent synchronized oscillations in prolactin gene promoter activity in living pituitary cells. Endocrinology142, 3255–3260 (2001). CASPubMedGoogle Scholar
- Shorte, S. L. et al. PRL gene expression in individual living mammotropes displays distinct functional pulses that oscillate in a noncircadian temporal pattern. Endocrinology143, 1126–1133 (2002). CASPubMedGoogle Scholar
- Berno, V. et al. Activation of estrogen receptor-α by E2 or EGF induces temporally distinct patterns of large-scale chromatin modification and mRNA transcription. PLoS ONE.3, e2286 (2008). PubMedPubMed CentralGoogle Scholar
- So, L. H. et al. General properties of transcriptional time series in Escherichia coli. Nature Genet.43, 554–560 (2011). CASPubMedGoogle Scholar
- Mortensen, K. I., Churchman, L. S., Spudich, J. A. & Flyvbjerg, H. Optimized localization analysis for single-molecule tracking and super-resolution microscopy. Nature Methods7, 377–381 (2010). CASPubMedPubMed CentralGoogle Scholar
- Brown, C. M. et al. Raster image correlation spectroscopy (RICS) for measuring fast protein dynamics and concentrations with a commercial laser scanning confocal microscope. J. Microsc.229, 78–91 (2008). CASPubMedPubMed CentralGoogle Scholar
- Digman, M. A., Dalal, R., Horwitz, A. F. & Gratton, E. Mapping the number of molecules and brightness in the laser scanning microscope. Biophys. J.94, 2320–2332 (2008). CASPubMedGoogle Scholar
- Sengupta, P. et al. Probing protein heterogeneity in the plasma membrane using PALM and pair correlation analysis. Nature Methods8, 969–975 (2011). CASPubMedPubMed CentralGoogle Scholar
- Robertson, G. et al. Genome-wide profiles of STAT1 DNA association using chromatin immunoprecipitation and massively parallel sequencing. Nature Methods4, 651–657 (2007). CASPubMedGoogle Scholar
- Lickwar, C. R., Mueller, F. & Lieb, J. D. Genome-wide measurement of protein–DNA binding dynamics using competition ChIP. Nature Protoc.8, 1337–1353 (2013). Google Scholar
- Hockensmith, J. W., Kubasek, W. L., Vorachek, W. R., Evertsz, E. M. & von Hippel, P. H. Laser cross-linking of protein–nucleic acid complexes. Methods Enzymol.208, 211–236 (1991). CASPubMedGoogle Scholar
- Nagaich, A. K. & Hager, G. L. UV laser cross-linking: a real-time assay to study dynamic protein/DNA interactions during chromatin remodeling. Sci. STKE256, L13 (2004). Google Scholar
- Becker, P. B., Ruppert, S. & Schutz, G. Genomic footprinting reveals cell type-specific DNA binding of ubiquitous factors. Cell51, 435–443 (1987). CASPubMedGoogle Scholar
- Koster, M., Frahm, T. & Hauser, H. Nucleocytoplasmic shuttling revealed by FRAP and FLIP technologies. Curr. Opin. Biotechnol.16, 28–34 (2005). PubMedGoogle Scholar
- Mazza, D., Abernathy, A., Golob, N., Morisaki, T. & McNally, J. G. A benchmark for chromatin binding measurements in live cells. Nucleic Acids Res.40, e119 (2012). CASPubMedPubMed CentralGoogle Scholar
- Sun, Y., Hays, N. M., Periasamy, A., Davidson, M. W. & Day, R. N. Monitoring protein interactions in living cells with fluorescence lifetime imaging microscopy. Methods Enzymol.504, 371–391 (2012). CASPubMedPubMed CentralGoogle Scholar
- Mazza, D., Ganguly, S. & McNally, J. G. Monitoring dynamic binding of chromatin proteins in vivo by single-molecule tracking. Methods Mol. Biol.1042, 117–137 (2013). CASPubMedGoogle Scholar
- Bustamante, C., Cheng, W. & Mejia, Y. X. Revisiting the central dogma one molecule at a time. Cell144, 480–497 (2011). CASPubMedPubMed CentralGoogle Scholar
Acknowledgements
The authors acknowledge careful reading of the manuscript and numerous suggestions by L. Grontved and D. Pressman, the US National Institutes of Health.